Fast, Flexible Ionic Transistors for Bioelectronic Devices
February 28, 2019 | Columbia EngineeringEstimated reading time: 5 minutes
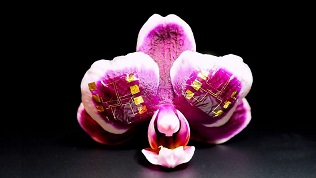
Many major advances in medicine, especially in neurology, have been sparked by recent advances in electronic systems that can acquire, process, and interact with biological substrates. These bioelectronic systems, which are increasingly used to understand dynamic living organisms and to treat human disease, require devices that can record body signals, process them, detect patterns, and deliver electrical or chemical stimulation to address problems.
Image Caption: IGT-based NAND and NOR gates conform to the surface of orchid petals (left). Scale bar, 1cm. Optical micrographs of NOR (upper right) and NAND (lower right) logic gates. Input (I1, I2) and output (O) configuration is indicated. Scale bar, 100 mm.
Transistors, the devices that amplify or switch electronic signals on circuits, form the backbone of these systems. However, they must meet numerous criteria to operate efficiently and safely in biological environments such as the human body. To date, researchers have not been able to build transistors that have all the features needed for safe, reliable, and fast operation in these environments over extended periods of time.
A team led by Dion Khodagholy, assistant professor of electrical engineering at Columbia Engineering, and Jennifer N. Gelinas, Columbia University Medical Center, Department of Neurology, and the Institute for Genomic Medicine, has developed the first biocompatible ion-driven transistor that is fast enough to enable real-time signal sensing and stimulation of brain signals.
(A) Schematic illustration of IGT cross-section and wiring diagram for device operation (upper). D-sorbitol creates an ion reservoir, maintaining mobile ions (green) that can travel within the channel; PEDOT-rich regions are shown in light blue and PSS lamellas in white (lower).
(B) Optical micrograph displaying the top view of an individual transistor. Scale bar, 20 µm. Inset shows a cross-section scanning electron microscopy image acquired at a tilt angle of 30°. Ion membrane (light red), channel (light blue) and Au contacts for gate (G) and source (S; beige) are visible. Scale bar, 5 µm.
The internal-ion-gated organic electrochemical transistor (IGT) operates via mobile ions contained within a conducting polymer channel to enable both volumetric capacitance (ionic interactions involving the entire bulk of the channel) and shortened ionic transit time. The IGT has large transconductance (amplification rate), high speed, and can be independently gated as well as microfabricated to create scalable conformable integrated circuits. In their study published today in Science Advances, the researchers demonstrate the ability of their IGT to provide a miniaturized, soft, conformable interface with human skin, using local amplification to record high quality neural signals, suitable for advanced data processing.
“We’ve made a transistor that can communicate using ions, the body’s charge carriers, at speeds fast enough to perform complex computations required for neurophysiology, the study of the nervous system function,” Khodagholy says. “Our transistor’s channel is made out of fully biocompatible materials and can interact with both ions and electrons, making communication with neural signals of the body more efficient. We’ll now be able to build safer, smaller, and smarter bioelectronic devices, such as brain-machine interfaces, wearable electronics, and responsive therapeutic stimulation devices, that can be implanted in humans over long periods of time.”
In the past, traditional silicon-based transistors have been used in bioelectronic devices, but they must be carefully encapsulated to avoid contact with body fluids—both for the safety of the patient and the proper operation of the device. This requirement makes implants based on these transistors bulky and rigid. In parallel, a good deal of work has been done in the organic electronics field to create inherently flexible transistors out of plastic, including designs such as electrolyte-gated or electrochemical transistors that can modulate their output based on ionic currents. However, these devices cannot operate fast enough to perform the computations required for bioelectronic devices used in neurophysiology applications.
Khodagholy and his postdoctoral research fellow George Spyropoulos, the first author of this work, built a transistor channel based on conducting polymers to enable ionic modulation, and, in order to make the device fast, they modified the material to have its own mobile ions. By shortening the distance that ions needed to travel within the polymer structure, they improved the speed of the transistor by an order of magnitude compared to other ionic devices of the same size.
Page 1 of 2
Suggested Items
Happy’s Tech Talk #34: Producibility and Other Pseudo-metrics
11/12/2024 | Happy Holden -- Column: Happy’s Tech TalkAs an engineer, I thrive on data, and one of my favorite forms is metrics. However, the one metric that has always challenged me is producibility. I define this as more than just passing a DRC in CAM, but the entire envelope of “simplicity of design,” “suitability for test,” and many more. Yet, producibility seemed to be different for different engineers and we had no clear way to establish and define producibility other than opinion. When I worked at HP, the company invested in a methodology called design for manufacturing and assembly using the GE/Hitachi Methodology and Dewhurst-Boothroyd software. Finally, I had a methodology that created a producibility score.
Flexible Thinking: Musings on High Density Interconnections
10/30/2024 | Joe Fjelstad -- Column: Flexible ThinkingPeople have been using high density interconnection (HDI) technology since the early 1980s, although it was not called HDI until the late 1990s. In the 1970s, ’80s, and early ’90s, engineers used HDI methods to develop hybrid circuits, which were later referred to as multichip modules (MCMs). These were arguably the first instantiation of heterogeneous interconnection technology, which has been the industry buzzword for almost a decade. These devices are a way of integrating multiple chips—both integrated circuits and discrete devices (resistors, capacitors, and inductors)—into a single package, typically using ceramic substrates with layers of insulation and metallic inks (often gold) and firing them at high temperatures.
It’s Only Common Sense: Want to Succeed? Stay in Your Lane
10/28/2024 | Dan Beaulieu -- Column: It's Only Common SenseIn today’s busy world, there are so many things going on, that it’s easy to get distracted. We live in an era of constant comparison and nonstop disruption. Everyone’s talking about the latest technology, the economy’s twists and turns, globalization, or how Company X is doing something you’re not. But here’s the thing: None of that matters if you’re not focused on your path. I’m going to say it straight: Stay in your lane. It’s the only way you’ll ever find success in business and life.
Book Excerpt: The Printed Circuit Designer’s Guide to... DFM Essentials, Ch. 1
10/25/2024 | I-Connect007The guidelines offered in this book are based on both ASC recommendations and IPC standards with the understanding that some may require adjustment based on the material set, fabricator processes, and other design constraints. This chapter details high-frequency materials, copper foil types, metal core PCBs, and the benefits of embedded capacitance and resistor materials in multilayer PCBs.
The Cost-Benefit Analysis of Direct Metallization
10/21/2024 | Carmichael Gugliotti, MacDermid AlphaCarmichael Gugliotti of MacDermid Alpha discusses the innovative realm of direct metallization technology, its numerous applications, and significant advantages over traditional processes. Carmichael offers an in-depth look at how direct metallization, through developments such as Blackhole and Shadow, is revolutionizing PCB manufacturing by enhancing efficiency, sustainability, and cost-effectiveness. From its origins in the 1980s to its application in cutting-edge, high-density interconnects and its pivotal role in sustainability, this discussion sheds light on how direct metallization shapes the future of PCB manufacturing across various industries, including automotive, consumer electronics, and beyond.