-
- News
- Books
Featured Books
- pcb007 Magazine
Latest Issues
Current IssueThe Hole Truth: Via Integrity in an HDI World
From the drilled hole to registration across multiple sequential lamination cycles, to the quality of your copper plating, via reliability in an HDI world is becoming an ever-greater challenge. This month we look at “The Hole Truth,” from creating the “perfect” via to how you can assure via quality and reliability, the first time, every time.
In Pursuit of Perfection: Defect Reduction
For bare PCB board fabrication, defect reduction is a critical aspect of a company's bottom line profitability. In this issue, we examine how imaging, etching, and plating processes can provide information and insight into reducing defects and increasing yields.
Voices of the Industry
We take the pulse of the PCB industry by sharing insights from leading fabricators and suppliers in this month's issue. We've gathered their thoughts on the new U.S. administration, spending, the war in Ukraine, and their most pressing needs. It’s an eye-opening and enlightening look behind the curtain.
- Articles
- Columns
- Links
- Media kit
||| MENU - pcb007 Magazine
Estimated reading time: 9 minutes
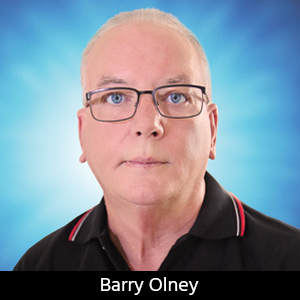
Beyond Design: Does Current Deliver the Energy in a Circuit?
Editor's note: Rick Hartley also contributed to this column.
In circuit theory, we learn that current flows in a closed loop, returning to the source via a conductor pair. This flow of current provides power to the load, and energy transfer occurs as described by Ohm’s law (P = VI). Conversely, in electromagnetics theory, we discover that current does not provide the power in a circuit, but rather the energy wave is guided by the conductor to power the load, according to Maxwell’s equations.
The flow of current in a PCB is essential for delivering energy and enabling devices to perform work but does current carry the energy? Why does an alternating current (AC) signal require a thicker conductor to provide higher power if the current is not in the carrier? There are many contradictions as to how energy flows in a circuit. To understand this, one must appreciate the difference between the lumped element view and that of distributed circuits. Ohm’s law provides a simplified approach to power transfer whereas Maxwell’s equations offer an interpretation of the entire distributed system. In this month’s column, we will try to clarify the perplexing aspect of AC/DC electrical theory.
Electromagnetic (EM) waves carry energy through their electric and magnetic fields. The larger the strength of these fields, the more work they can do and the greater the energy the wave carries. At high frequencies, the diameter of a conductor affects how efficiently it absorbs energy from electromagnetic waves. Larger diameters allow for better energy absorption, while smaller diameters limit energy transfer. The wave energy is determined by its amplitude. The amplitude of an electromagnetic wave refers to the maximum field strength of its electric and magnetic fields. The energy carried by an electromagnetic wave is directly proportional to the square of its amplitude. In other words, if the amplitude of a wave doubles, its energy increases by four times. This relationship holds true for all types of electromagnetic waves (Figure 1).
When electromagnetic waves encounter a conductor (such as a PCB trace and its reference line, typically a ground conductor), they induce oscillating currents in the conductors due to the fluctuating electric and magnetic fields. A pair of associated conductors, usually a signal line and reference line, are used to move energy from point A to point B. These induced currents themselves emit electromagnetic waves at the same frequency, which can either constructively or detrimentally interfere. If the conductor has a small diameter (compared to the wavelength of the incident wave), the induced currents are limited, and the energy absorption is reduced. Conversely, a larger conductor diameter allows for more efficient energy absorption.
It’s essential to note that the speed of energy/signal traveling down a conductor is actually the speed of the electromagnetic wave, not the movement of electrons. The signal travels on this electromagnetic carrier wave. The EM wave traveling along (guided by) the copper trace forms a type of waveguide. The propagation of the wave is affected by the interaction with the material(s) in and surrounding the dielectric, caused by the presence of electric charge carriers, interacting with the electric field component, and magnetic dipoles, interacting with the magnetic field component. The energy/signal flows overwhelmingly outside the electrical conductor. The purpose of the conductor is thus not to conduct energy, but to guide the energy-carrying wave.
The electromagnetic fields do not move through space. It is the electromagnetic energy that moves. The electric field of a conductor extends to the return conductor, which is usually closely coupled to the signal trace. At greater distances, the aggregate field can be approximated by the differential field between the conductor and the return conductor, which tends to cancel. When you minimize the return loop in a circuit, it helps reduce electromagnetic radiation.
When a DC step voltage is applied to a transmission line, the electron drift velocity will increase in speed proportionally to the strength of the electric field. However, electron drift speed is very slow; drift velocity is about 4 Km/hr. AC voltages cause no net movement. The electrons oscillate back and forth in response to the alternating electric field, over a distance of a few micrometers.
The velocity of propagation of an EM wave is only limited by the dielectric properties of the surrounding materials. EM waves travel at the speed of light in a vacuum or air but are slowed down as they enter a dielectric. The relative permeability or dielectric constant (), of the surrounding materials, impacts the velocity of propagation (v) at the speed of light (c).
Equation 1
Therefore, FR-4 material (with Dk = 4) in a stripline configuration slows the propagation speed and decreases the wavelength of the electromagnetic wave by about half. But that all depends on the exact dielectric constant of the combined surrounding materials.
It is important to note that this speed applies to all electromagnetic waves, including direct current (DC) signals. Even though DC signals are typically associated with steady currents, their electromagnetic fields still propagate at the same speed as other electromagnetic waves. So, whether it’s a radio wave, visible light, or a DC signal, they all travel at this universal speed. When a DC voltage is applied to a transmission line, the particles move, generating a magnetic field, which in turn creates an EM wave along the line. Signals do not travel as electrons or charge movement; it would be way too slow to be useful. Signals travel at a fraction of light speed because it's the EM wave that travels.
Electromagnetic waves are generated by the acceleration of charged particles. In the case of DC, a constant electric field creates the flow of electrons through a conductor pair, creating a constant magnetic field. This magnetic field then generates an electromagnetic wave. The strength of the DC current does not affect the frequency of the electromagnetic wave, but it does affect the amplitude or intensity of the wave. A stronger current will result in a stronger electromagnetic wave with a higher amplitude and thus more energy.
When a DC voltage is connected to a load (via a PCB trace), a transient current flows through the conductors. This transient current is actually an alternating current and behaves like a propagating electromagnetic wave. It generates fluctuating electric and magnetic fields as it travels along the conductor. If the impedance of the transmission line is not matched to the source and the load, it will reflect, creating overshoot and undershoot as in Figure 2.
It is important to understand that fluctuating DC cannot exist; anything that fluctuates is actually AC. As designers, we often use the term DC rather too loosely. At the instant of connection of a DC voltage to a load, a transient current flows through the conductors (PCB traces), charging up their distributed capacitances. This transient current is AC and is a propagating EM wave that generates fluctuating EM fields (magnetic and electric) as it travels along the transmission line. Afterward, the current is DC and the EM fields become static, not fluctuating.
Electric and magnetic fields are present around any electrical circuit, whether it carries AC or DC electricity. Since DC is static and AC varies in direction, fields from DC and AC sources have significant differences. Static fields, for example, do not induce currents (crosstalk) in stationary objects, while AC fields do. Static magnetic fields do not vary over time and thus do not have a frequency (0 Hz). Therefore, DC signals do not exhibit the skin effect, where current tends to flow only in the surface of a conductor. As the frequency increases, current is delegated to the surface.
Charges do not move in a conductor unless there is an electric field to push them along. In a vacuum, electrons are unimpeded and accelerate across the available space. They give up their energy on impact at the end of their journey. In a dielectric, the moving charges constantly collide with atoms, creating thermal energy (heat). On average, they attain a fixed velocity. This steady flow of charge is called a direct current. A direct current that flows in a conductor flows uniformly through the entire conductor cross-section (not only on the surface).
In a DC circuit, energy flows due to the interplay of electric and magnetic fields. The energy transfer occurs through the interplay of the electric field (due to the presence of charged particles) and the magnetic field (due to the motion of charged particles). It is not current that delivers the energy to the load, but rather electrons moving through the copper create both fields, facilitating the energy transfer.
Designers often mislead themselves when they talk about a DC voltage rail. It tends to suggest that the currents that ICs draw from the DC rail are DC currents, when in fact, with modern digital electronics, they are actually quite powerful high-frequency currents plus a DC component, which is why we must be careful to decouple our power supply rails properly to control emissions. Thicker traces reduce the skin effect and minimize losses at AC, while a thicker trace at DC allows for freer movement of charged particles and thus reduces emitted heat.
Key Points
- One must appreciate the difference between the lumped element view and that of distributed circuits.
- At high frequencies, the diameter of a conductor affects how efficiently it absorbs energy from electromagnetic waves. A larger conductor diameter allows for more efficient energy absorption.
- If the conductor has a small diameter (compared to the wavelength of the incident wave), the induced currents are limited, and the energy absorption is reduced.
- The energy carried by an electromagnetic wave is directly proportional to the square of its amplitude.
- Energy/signal traveling down a conductor is actually the speed of the electromagnetic wave, not the movement of electrons.
- The EM wave traveling along (guided by) the copper trace forms a type of waveguide.
- The electric field of a conductor extends to the return conductor, which is usually closely coupled to the signal trace.
- Even though DC signals are typically associated with steady currents, their electromagnetic fields still propagate at the same speed as other electromagnetic waves.
- When a DC voltage is applied to a transmission line, it generates a magnetic field, which in turn creates an EM wave along the line.
- In the case of DC, a constant electric field creates the flow of electrons through a conductor pair, creating a constant magnetic field. This magnetic field then generates an electromagnetic wave.
- Electric and magnetic fields are present around any electrical circuit, whether it carries AC or DC electricity.
- It is not current that delivers the energy to the load, but rather electrons moving through the copper create EM fields, facilitating the energy transfer.
Resources
- Beyond Design: “The Wavelength of Electromagnetic Energy” by Barry Olney
- Fast Circuit Boards, by Ralph Morrison
- “Physics Stack Exchange,” Physics Forum
- Wikipedia: Speed of electricity
- “How are DC signals perceived as EM waves?” by Keith Armstrong
- University Physics Vol 2, Samuel J. Ling, et al
Rick Hartley is the principal engineer at R Hartley Enterprises and has been in the industry for over 50 years. He is one of the primary consultants for PCB manufacturing and design companies. Rick has also conducted classes worldwide on EMI, signal integrity, and various other electrical topics for the last 30 years.
This column originally appeared in the July 2024 issue of Design007 Magazine.
More Columns from Beyond Design
Beyond Design: The Metamorphosis of the PCB RouterBeyond Design: Radiation and Interference Coupling
Beyond Design: Key SI Considerations for High-speed PCB Design
Beyond Design: Electro-optical Circuit Boards
Beyond Design: AI-driven Inverse Stackup Optimization
Beyond Design: High-speed Rules of Thumb
Beyond Design: Integrated Circuit to PCB Integration
Beyond Design: Termination Planning