-
- News
- Books
Featured Books
- pcb007 Magazine
Latest Issues
Current IssueIn Pursuit of Perfection: Defect Reduction
For bare PCB board fabrication, defect reduction is a critical aspect of a company's bottom line profitability. In this issue, we examine how imaging, etching, and plating processes can provide information and insight into reducing defects and increasing yields.
Voices of the Industry
We take the pulse of the PCB industry by sharing insights from leading fabricators and suppliers in this month's issue. We've gathered their thoughts on the new U.S. administration, spending, the war in Ukraine, and their most pressing needs. It’s an eye-opening and enlightening look behind the curtain.
The Essential Guide to Surface Finishes
We go back to basics this month with a recount of a little history, and look forward to addressing the many challenges that high density, high frequency, adhesion, SI, and corrosion concerns for harsh environments bring to the fore. We compare and contrast surface finishes by type and application, take a hard look at the many iterations of gold plating, and address palladium as a surface finish.
- Articles
- Columns
Search Console
- Links
- Media kit
||| MENU - pcb007 Magazine
Estimated reading time: 7 minutes
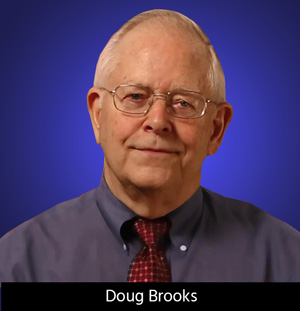
Contact Columnist Form
What Does Voltage Refer To, and Why Do We Care?
Here is today’s multiple-choice quiz. I am betting many of you do not get the right answer!
Figure 1 shows a 1.5 volt battery.
Q: What is the voltage at point A.
- 1.5 volts.
- Since there is not a compete circuit (current loop), there is no voltage at point A.
- The question is not correct.
Well, answer b. is flat wrong. Current cannot flow unless there is a closed loop (see Note 1) but voltage can exist without a closed loop. But answer a. is not correct, either. The correct answer is c. The question is wrong! Voltage is not a point concept. Voltage does not occur at a point (even though we use that expression all the time.) Voltage occurs between two points. So the correct question would be “What is the voltage at point A with respect to (some other point). For example, the voltage between points A and B would be 1.5 volts. Later on in this article you will begin to appreciate the importance of this. It is very important in things like power distribution systems (power supply distribution.)
Figure 1. What is the voltage at point A?
Definition of Voltage
If you do a search for voltage on the Web, you will find lots of definitions. For our purposes here, we can consider it to be the force that causes current to flow. Since current is the flow of electrons (again, see Note 1), and since electrons are negatively charged, we can consider voltage to be the difference in charge between two points. The more negatively charged point repels electrons and the more positively charged point attracts electrons, thus resulting in the current flow. (See Note 2 for a discussion of which direction current flows.)
Visualizing a difference in charge is intuitive if we think of a battery. One terminal is positively charged with respect to the other terminal because of the chemical reactions internal to the battery. It is less intuitive if we think of what is at the electrical socket in our walls. The driving potential at the socket originates at a generator somewhere. For me, near Seattle, it is probably in a generator at a hydroelectric dam on one of the many rivers in our state.
From a practical standpoint, we tend to take the current generated by a generator, transform it, rectify it, and charge capacitors with it. That provides the power supply systems for the electronic equipment we design. In the end, a charged capacitor acts very much like a battery. So, in the equipment (and PCBs) we design, the difference in charge originates either from a battery or from a power supply that creates something that looks very much like a battery (a charged bulk capacitor).
Importance of a Reference
Figure 2 is slightly modified from Figure 1. There is now a connection between A and C. The voltage between A and B is still 1.5 volts. But the voltage between A and D is zero volts. Since there is no current flowing in this figure, there is no voltage drop across the resistor. Points A and D are at the same potential.
But what about the voltage between points A and E (shown as a reference plane)? We don’t know what that voltage is because we don’t know what the potential is on the reference plane with respect to any other points in the figure.
Figure 2. Battery partially connected to a resistor.
Finally, consider the circuit in Figure 3. Think of this as a battery and a resistor on the top layer of a PCB. There is a trace between Points A and C, and Pads B and D are connected to the underlying “reference” plane through vias. Now there is a closed circuit and current does flow.
We can intuitively see that the voltage between points A and E is 1.5 volts, as is the voltage between points C and E. In fact, if I now asked what the voltage is at point A, we would interpret that to mean the voltage between points A and E. And that understanding often gets us into trouble! Here’s why.
Figure 3. Two components on a PCB.
All References are not Equal!
I am going to exchange the components in Figure 3 (a battery and a resistor) for two integrated circuit gates, as shown in Figure 4. I have omitted a few things (such as the positive power connections), but the trace connecting the two circuits (between points A and C) and the connections to the reference plane are analogous to those in Figure 3. Except, in the real world, connections to the plane have inductance, as shown in Figure 4.
Figure 4. Circuits on a PCB.
Now, when I ask what the voltage is at point A, what exactly do I mean? The output of the driver IC (point A) is actually referenced to its reference (on the chip) at point B. The voltage at point C, the input to the receiving IC, is actually referenced to its reference on its chip at point D. But we, as board, circuit, and system designers, think of the reference plane, E, as the reference. These points are not always equal, even though we like to think so. So if I think about an output of a logical zero (say 0.3 volts) at the output of the driver, do I mean the voltage at point A is 0.3 volts between the output and point E, point B, or point D? It can make a difference.
The biggest difference is likely due to switching noise voltage across the inductor, L1. The noise voltage across this inductor is (approximately) given by
V = L x di/dt , whereV is the noise voltageL is the inductancedi is the switched current (you can think of it as delta-i, or the change in current), anddt is the rise- or fall-time (the change in time).
In typical PCB applications L is extremely small, say on the order of 5 x 10-9. But so is dt. When we are dealing in rise and fall times less than a nanosecond, dt can be smaller than L. Let’s assume that dt = 5 x 10-10 (500 picoseconds). If we used these two values (5 nanoHenrys and 500 picoseconds), the noise voltage becomes
V = di x 10 !
Now di is also probably pretty small – unless we are dealing with a BGA in which maybe 10,000 circuits are all switching at the same point in time! Then the input voltage at point C (with respect to its reference, point D) is the logical zero voltage at point A (with respect to its reference at point B), PLUS the noise generated across the inductor L1. (Under the conditions we have specified that we can assume the voltage across L2 is negligible.) If the noise voltage is large enough, the receiver might not be able to tell whether the signal on Trace AC is a logical zero or a logical one.
The noise voltage across the inductor L1 is often referred to as “ground bounce,” a major source of signal integrity problems. Integrated circuit manufacturers put a lot of thought and effort into designing their chips with the minimization of ground bounce, and the tolerance for noise margins, in mind. Then we as board designers put thought and effort into designing low inductance pads and vias to minimize contributors to the total inductance, L1. Finally, we employ bypass capacitor and planar capacitance strategies to help control switching noise (of which ground bounce is one of the major contributors.) These are all topics covered in signal integrity discussions.
Of Importance in this Discussion
Recognize that understanding the voltage reference for the various signals we are dealing with is an important issue in being able to design solutions on our boards.
Notes:
- See my column “What is Current and Why Do We Care” available at http://www.pcbdesign007.com/pages/zone.cgi?a=75263&artpg=1
- I believe it was Benjamin Franklin who said that current flows from positive to negative. And we have believed that ever since. But if current is the flow of electrons, and electrons flow from negative to positive, then doesn’t current flow from negative to positive instead? It turns out that in terms of analyzing a circuit, it makes absolutely no difference which direction you believe (the fundamental nature of) current flows. The situation is symmetrical and you get the same answer either way. Some even suggest that if negative charges are flowing in one direction, we can think of positive charges flowing in the other direction (even though there aren’t any positively charges particles flowing at all!). So even though we know that electrons flow from negative to positive, we still use the traditional definition that current flows from positive to negative without any problems. The only exception I have encountered in my career is when we are analyzing the physical flow of current (electrons) in semiconductor junctions. There it really does matter which direction the electrons are flowing.
Douglas Brooks has an MS/EE from Stanford University and a Ph.D. from the University of Washington. He has spent most of his career in the electronics industry in positions of engineering, marketing, general management, and as CEO of several companies. He has owned UltraCAD Design Inc. since 1992. He is the author of numerous articles in several disciplines, and has written articles and given seminars all over the world on Signal Integrity issues since founding UltraCAD. His book, Printed Circuit Board Design and Signal Integrity Issues was published by Prentice Hall in 2003. Visit his Web site at www.ultracad.com.
More Columns from Brooks' Bits
Brooks' Bits: Internal Trace Temperatures—More Complicated Than You ThinkBrooks' Bits: Electromagnetic Fields, Part 3 - How They Impact Coupling
Brooks' Bits: Electromagnetic Fields, Part 2: How They Impact Propagation Speed
Brooks' Bits: How Electromagnetic Fields Determine Impedance, Part 1
Trace Currents and Temperature, Part 4: Via Heat
Trace Currents and Temperature, Part 3: Fusing Currents
Trace Currents and Temperature, Part 1: The Basic Model
The Skinny on Skin Effect, Part 3: Crossover Frequency