-
- News
- Books
Featured Books
- pcb007 Magazine
Latest Issues
Current IssueInner Layer Precision & Yields
In this issue, we examine the critical nature of building precisions into your inner layers and assessing their pass/fail status as early as possible. Whether it’s using automation to cut down on handling issues, identifying defects earlier, or replacing an old line...
Engineering Economics
The real cost to manufacture a PCB encompasses everything that goes into making the product: the materials and other value-added supplies, machine and personnel costs, and most importantly, your quality. A hard look at real costs seems wholly appropriate.
Alternate Metallization Processes
Traditional electroless copper and electroless copper immersion gold have been primary PCB plating methods for decades. But alternative plating metals and processes have been introduced over the past few years as miniaturization and advanced packaging continue to develop.
- Articles
- Columns
Search Console
- Links
- Media kit
||| MENU - pcb007 Magazine
Estimated reading time: 6 minutes
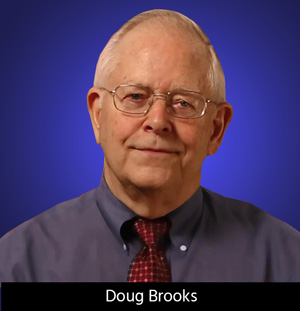
Contact Columnist Form
Rise Time vs. Frequency: What's the Relationship?
The frequency of a waveform is the number of times it repeats (cycles) in one second. A sine wave of 100 Hz (many years ago we called it “cycles-per-second,” which actually had an intuitive meaning!) repeats itself (goes through its cycle) 100 times each second.
The rise time (or alternatively the fall time) of a signal is defined as the time it takes the waveform to transition from one peak level to the other. We usually specify the rise time as the time between the 10% and 90% points in this transition (see Figure 1), but some spec sheets will specify it as the time between the 20% and 80% points.
Figure 1. Rise time of a waveform and the period of an underlying sine wave.
You might ask: Why do we refer to the 10% to 90% range of amplitude for rise time and not the 0% to 100%? Or, why do some use the 20% to 80% measure for rise time? The answer may be somewhat less than satisfying, but it goes like this: Almost all switching devices follow a switching pattern similar to that shown by the rising line in Figure 1, but they may differ significantly in the very first or last portions of their switching pattern.
For example, one type of device may reach 100% very quickly, but another device may roll off as it approaches the 100% level, and actually may not reach 100% for several moments (we sometimes say it may approach 100% asymptotically). Switching devices might have quite different switching patterns in the very beginning or ending stages of their switching range, but usually all have quite similar patterns during their main transition.
And, the 10% and 90% points may more closely resemble the switching “threshold” levels of the associated logic devices. Therefore, we use the 10% to 90% points of the switching range for the rise time measure in order to compare “apples to apples.” Sometimes others may use the 20% to 80% range because the beginning and ending parts of the switching range are a little “broader” than other devices, because the logic switching thresholds are more closely spaced, or because they are engaging in “specsmanship” (the 20% to 80% range is shorter and therefore faster).
There is no necessary relationship between rise time and frequency (See Figure 2). There is an exception to this rule in the very special case of a well formed signal. From a practical standpoint for those of us in the PCB design business, a sine (or cosine) waveform is the one (I believe) exception. In this case, the rise time is approximately 1/3 of the period of the waveform (the period being the inverse of the frequency, see Figure 1.) Thus, a 100 Hz sine wave has a rise time of 1/3 of the period (1/100 of a second), or about 3.3 milliseconds (see Note 1).
Figure 2. The sine and square waves have the same frequency but much different rise times.
But consider a square wave whose frequency is 100 Hz. If it is an “ideal” waveform it will have zero rise and fall times. If the rise and fall times are linear (but not zero), then the waveform is not strictly square, but it is trapezoidal. In any event, we can know nothing about the rise time of an approximately square waveform without at least some other information.
These two measurements, frequency and rise time, can be quite important to us, but in quite different ways. Frequency is inherently related to timing and information. This has implications for the sequencing of operations (as a clock signal controls the sequence of a controller circuit). It also can be directly related to information – an audio signal is a perfect example. As long as frequencies remain undistorted, we can predict what will happen in the various circuits we design.
Rise time is related to how quickly changes can/do happen. A circuit must have a fast enough rise time to accommodate the signal being processed. If it does not, information in the waveform or circuit timing may be lost or distorted. But here is the clincher: a circuit does NOT have to have a faster rise time than is required by the waveform. Faster is not necessarily better.
In one sense, we have opposite feelings about these measures. Faster frequencies are good. Just ask any computer operator! But faster rise times are bad. The reason is that a very large number of signal integrity issues are caused by or exacerbated by rise time effects. In a very general sense, signal integrity issues can fall into four categories (see Note 2):
- Radiated effects (e.g., EMI, crosstalk)
- Inductance effects (e.g., ground bounce)
- Reflection issues (related to transmission lines)
- Resistance as a function of frequency (skin effect, dielectric losses)
Rise times are intimately related to the first three of these.
- Radiation effects, in general terms, increase with the cube of the frequency, i.e., with f3. The faster the rise time of a circuit, the wider the bandwidth and the higher the frequencies that the circuit can effectively transmit around the board. Some of these frequencies may be unwanted (and harmful) noise harmonics generated somewhere in our circuit. That is why we like to have circuit rise time capabilities no higher than is necessary to handle the signals on our boards.
- Rise time is the change of current with respect to time, or ∆i/ ∆t. The calculus expression of this is di/dt. The faster the rise time, the smaller is dt. There is inductance everywhere in the power supply distribution system. The noise voltage spike across any inductance, L, is L*di/dt. So smaller dt results in larger noise voltage spikes (one of the sources of ground bounce.)
- A signal trace longer than the “critical length” can create reflection issues in our circuits. The solution to reflection problems is controlled impedance traces and proper trace terminations. The critical length is proportional to rise time (see Note 3). So, faster rise times than necessary can result in shorter critical lengths and greater reflection issues.
It is very important for designers to focus on these two parameters, frequency and rise time, separately. That way, we can pay attention to desired circuit performance, on the one hand, and destructive signal integrity issues on the other.
Notes
- Text books may say that a more precise relationship is Tr = 1/f*π, where Tr is the rise time and f is the frequency in Hz. This is, in fact, approximately equal to 1/3 the period.
- See my article “A History of Signal Integrity: One Man's Perspective” available here.
- In general, the critical length for a trace is that length for which the propagation of the signal down the trace and back again is equal to the rise time. Shorter (faster) rise times mean shorter critical lengths.
Douglas Brooks has an MS/EE from Stanford University and a Ph.D. from the University of Washington. He has spent most of his career in the electronics industry in positions of engineering, marketing, general management, and as CEO of several companies. He has owned UltraCAD Design Inc. since 1992. He is the author of numerous articles in several disciplines and has written articles and given seminars all over the world on Signal Integrity issues since founding UltraCAD. His book, Printed Circuit Board Design and Signal Integrity Issues, was published by Prentice Hall in 2003. Visit his website at ultracad.com.
More Columns from Brooks' Bits
Brooks' Bits: Internal Trace Temperatures—More Complicated Than You ThinkBrooks' Bits: Electromagnetic Fields, Part 3 - How They Impact Coupling
Brooks' Bits: Electromagnetic Fields, Part 2: How They Impact Propagation Speed
Brooks' Bits: How Electromagnetic Fields Determine Impedance, Part 1
Trace Currents and Temperature, Part 4: Via Heat
Trace Currents and Temperature, Part 3: Fusing Currents
Trace Currents and Temperature, Part 1: The Basic Model
The Skinny on Skin Effect, Part 3: Crossover Frequency