-
- News
- Books
Featured Books
- pcb007 Magazine
Latest Issues
Current IssueVoices of the Industry
We take the pulse of the PCB industry by sharing insights from leading fabricators and suppliers in this month's issue. We've gathered their thoughts on the new U.S. administration, spending, the war in Ukraine, and their most pressing needs. It’s an eye-opening and enlightening look behind the curtain.
The Essential Guide to Surface Finishes
We go back to basics this month with a recount of a little history, and look forward to addressing the many challenges that high density, high frequency, adhesion, SI, and corrosion concerns for harsh environments bring to the fore. We compare and contrast surface finishes by type and application, take a hard look at the many iterations of gold plating, and address palladium as a surface finish.
It's Show Time!
In this month’s issue of PCB007 Magazine we reimagine the possibilities featuring stories all about IPC APEX EXPO 2025—covering what to look forward to, and what you don’t want to miss.
- Articles
- Columns
Search Console
- Links
- Media kit
||| MENU - pcb007 Magazine
Estimated reading time: 6 minutes
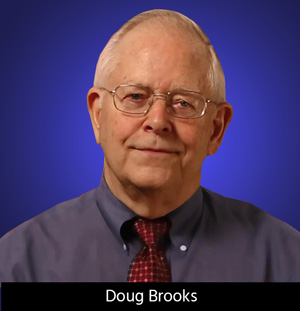
Contact Columnist Form
Crosstalk: Why It's Difficult to Understand, Part 1
Many people find crosstalk difficult to understand. Perhaps this is because there are a few unusual characteristics about it:
1: It has two different fundamental causes
2: These causes generate two different signals
3: These two signals flow in opposite directions
4: These signals can interact with each other
5: These two signals have significantly different shapes
6: These shapes behave differently as a function of coupled length, and
7: Neither shape resembles the “aggressor” signal that caused the crosstalk in the first place!
Aside from these few minor points, crosstalk is pretty easy to understand! So let’s see if we can make some sense out of all of this. First, to have crosstalk on our circuit boards we must have at least two traces. One of these carries a driving signal that is going to do the coupling. We call this the aggressor trace. The other trace(s) receives the coupled signal (crosstalk). We call this the victim trace. It is possible in practical circuits for a trace to be both an aggressor trace and a victim trace at the same time, although we usually analyze these effects separately.
The degree of coupling is related to the length over which the coupling occurs. Traces that are perpendicular to each other have a very short coupled length. Crosstalk is usually not an issue with traces that are routed perpendicular to each other. Therefore, crosstalk relates only to traces that are (more or less) parallel to each other and relatively close to each other. The length over which this is true is called the coupled length. The coupled length may be only a portion of the total length of a trace.
Two Fundamental Causes
Crosstalk is created when a signal on one wire or trace couples into another wire or trace. Since DC signals do not couple, we know right away that crosstalk is an AC effect. It is the electromagnetic field around the wire or trace that causes the coupling (see Note 1.)
The electromagnetic field has two components: An electric field and a magnetic field. These two fields are very closely related, but still different, with different effects. Think of the electric field as causing an electric, a charge, or a capacitive coupling effect. This effect is caused by the electric charge (the electrons) component of the signal. The magnetic field is created any time a current flows down a trace. A changing current causes a changing magnetic field. A changing magnetic field causes magnetic, or inductive, coupling into an adjacent trace (see Note 2.) It is the changing nature of the aggressor signal that is responsible for the crosstalk coupling.
So, we have two independent (crosstalk) signal components coupled into the victim trace, a charge component and a magnetic component. This takes care of point 1, above.
Two Different Signals
Recall that current is the movement (flow) of electrons (See Note 3.) An electron is a charged particle. Like charges repel and unlike charges attract. If you move a charged particle onto one plate of a capacitor, for example, a like charge is repelled from the other plate of the capacitor. Similarly, if you move a charged particle down a trace, like charges on an adjacent trace are repelled away from that charged particle. The like charges are repelled in all directions, but since they are constrained to the conductive trace, they move in both directions away from the particle that is repelling them. These repelled charges become a current that flows in both directions away from the charge that is repelling them.
This illustrates two important points. First, crosstalk is what we call a point concept. That is, it occurs at a single point along the trace (or at multiple points simultaneously). We can (and do) analyze multiple points independently. We call this point the “coupled point.” As the driving signal propagates down the trace, the coupled point moves along with it. So the driving signal couples into the victim trace at one point in one instance of time, and at a subsequent point at a later instance of time.
Secondly, this charge-coupled current flows in both directions away from the coupled point. Since (in general) perpendicular traces don’t result in crosstalk (only parallel traces do), these two directions are either in the same direction as the aggressor signal or in the opposite direction. Coupled signals that flow in the same direction as the aggressor signal are called forward crosstalk and signals that flow in the reverse direction are called backward crosstalk.
There is also an inductively coupled crosstalk signal. This always flows in the opposite direction of the current that causes it (i.e., the aggressor signal.) In a sense, this inductively coupled signal acts exactly like the coupled secondary current in a transformer.
So we have now taken care of points 2 and 3 listed earler. The two coupling effects (capacitive and inductive) create two independent currents. One of these currents flows in the backward direction and one flows in both directions (forward and backward) away from the point of coupling.
Interaction
Independent currents are additive. In the forward direction we have a capacitively coupled current that flows in the same direction as the aggressor signal and an inductively coupled current that flows in the opposite direction to the aggressor signal. These are approximately the same magnitude and tend to cancel each other out. In fact, under certain conditions they do exactly cancel each other out.
If the environment around the traces is homogeneous (i.e., uniform), the two components in the forward direction exactly cancel and there is no forward crosstalk current. The conditions in a stripline environment (with uniform dielectric coefficients) provide such an environment. In a stripline environment there is effectively no forward crosstalk current to worry about.
In a microstrip environment the conditions are not homogeneous. For example, there is a dielectric underneath the traces and solder coat, conformal coating and air above the traces. In a microstrip environment the two forward crosstalk components do not cancel, and there can be a forward crosstalk current generated by the coupling. Nevertheless, the two components tend to cancel, and the resulting forward crosstalk current is usually small. In general, we don’t worry about forward crosstalk currents on our circuit boards unless the coupling length is unusually long or there are some very special conditions involved.
In the backward direction the two crosstalk components add together, combining to create a backward crosstalk current. If we have a crosstalk problem on a circuit board, it is usually caused by backward crosstalk.
This takes care of point 4, above. In Part 2 of this series we will talk about the different shapes that characterize forward and backward crosstalk, and why crosstalk can be difficult to analyze when troubleshooting circuits.
Notes
1. While not strictly correct, it is not too wrong to say that if the coupled trace is close by we have crosstalk, if it is further away we have EMI. Both effects are caused by electromagnetic coupling, which is itself caused by the electromagnetic field around the aggressor trace.2. This is Faraday’s Law of Magnetic Induction.3. See my column “What is Current And Why Do We Care?”
Douglas Brooks has an MS/EE from Stanford University and a Ph.D. from the University of Washington. He has spent most of his career in the electronics industry in positions of engineering, marketing, general management, and as CEO of several companies. He has owned UltraCAD Design Inc. since 1992. He is the author of numerous articles in several disciplines, and has written articles and given seminars all over the world on Signal Integrity issues since founding UltraCAD. His book, Printed Circuit Board Design and Signal Integrity Issues was published by Prentice Hall in 2003. Visit his Web site at www.ultracad.com.
More Columns from Brooks' Bits
Brooks' Bits: Internal Trace Temperatures—More Complicated Than You ThinkBrooks' Bits: Electromagnetic Fields, Part 3 - How They Impact Coupling
Brooks' Bits: Electromagnetic Fields, Part 2: How They Impact Propagation Speed
Brooks' Bits: How Electromagnetic Fields Determine Impedance, Part 1
Trace Currents and Temperature, Part 4: Via Heat
Trace Currents and Temperature, Part 3: Fusing Currents
Trace Currents and Temperature, Part 1: The Basic Model
The Skinny on Skin Effect, Part 3: Crossover Frequency