Estimated reading time: 6 minutes
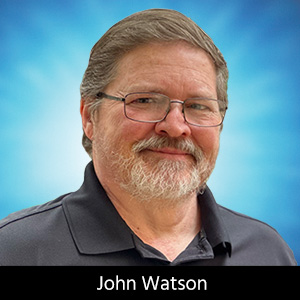
Elementary, Mr. Watson: The Gooey Centers of Hybrid PCB Designs
Anyone who knows me knows that I have a special relationship with food. Taking a twist on the words of Will Rogers, "I never met a buffet I didn't like." A balanced diet, to me, means having cupcakes in both hands.
One of my favorite foods is cheeseburgers. One of my colleagues at Palomar College recently invited me out for a meal, and we ended up in a burger place in San Diego. I wanted to go the conventional and safe route: a double-patty cheeseburger. My friend insisted that I try the restaurant’s special: a peanut butter cheeseburger. “Really?” I told him. “What an interesting combination.” But he insisted I try it, and since I consider myself an adventurous person, I gave it a go.
Let’s just say it only took one bite, and all my concerns floated away into a cloud of deliciousness: the contrast between sweet and savory, and the texture of the peanut butter's smoothness added a creamy contrast to the juicy, meaty texture of the burger. The peanut butter adds a layer of richness and depth, making each bite feel more indulgent. The subtle nutty taste complemented the beef and cheese without overpowering it. As you can probably tell, it was a great experience, and only deepend my special relationship with food.
In my PCB design course, I have used my cheeseburger analogy to teach the structure of a PCB design stack. When it comes to every stackup, there are four factors to consider:
- The number of layers in a PCB determines its complexity and functionality. PCBs can range from single-layer boards to multi-layer designs, from two to over 100 layers in high-end applications. Single-layer PCBs are simple, affordable, and typically used in basic electronics. Multi-layer boards, such as four, six, or eight layers, allow for more complex routing, higher component density, and better signal integrity, making them ideal for advanced electronics like smartphones and computers.
- The number and types of planes (power and/or ground) play a crucial role in PCB design. Ground planes provide a stable reference for circuit signals and reduce noise, while power planes distribute power efficiently across the board. Most multi-layer PCBs contain at least one ground plane and one power plane. In high-speed designs, multiple ground planes may be used to improve signal integrity and minimize electromagnetic interference (EMI).
- The ordering or sequence of the layers is where I see many novice designers make their mistakes. A multi-layer PCB is critical for performance, signal integrity, and manufacturability. Typically, outer layers are used for component placement and routing signals, while internal layers are reserved for power and ground planes and additional signal routing. For example, a common four-layer PCB sequence includes two outer signal layers, with an internal ground plane and a power plane in between.
- The spacing between PCB layers, known as the dielectric thickness, affects signal integrity, impedance, and thermal performance. Tighter spacing improves electrical performance but increases manufacturing complexity. Typical layer spacing ranges from 0.1 mm to 0.5 mm, depending on design requirements, ensuring proper insulation, reduced crosstalk, and controlled impedance for high-speed signals.
Just like in my analogy of a doube cheeseburger, there are two types of PCB design structures:
First is the foil buildup in PCB fabrication, which refers to the layering of thin copper foil sheets onto the board's substrate. These copper layers are laminated with dielectric materials and then etched to form conductive traces. The buildup process determines the board's copper thickness, influencing current-carrying capacity, heat dissipation, and overall performance. The process takes a core (in the center) and builds the PCB structure outward using the pre-preg and foil. What do we have here? It's a single patty cheeseburger with the patty representing the core.
The second type of PCB stackup is the core method. The core method in PCB stackup involves using a pre-manufactured, rigid core with copper foil on both sides as the foundation. Additional layers are added by bonding pre-preg (fiberglass with resin) and copper foil to the core through lamination. This method provides stability and helps build multi-layer PCBs efficiently. That is a double patty burger with the works.
This is a conventional, go-to PCB design and what you'll see mostly in your PCB design career—until that one day when someone suggests some peanut butter on your burger, and it turns your entire world upside down.
In this analogy, the peanut butter symbolizes specialty materials, like Rogers, ceramic, or Teflon-based laminates, often added to a hybrid PCB. These materials are chosen for specific properties that FR-4 lacks, such as low dielectric loss or higher thermal stability. Just as peanut butter brings a rich, unexpected flavor and texture to your cheeseburger, these advanced materials bring specific benefits, like better signal integrity for high-frequency applications or improved thermal management, which the FR-4 can't provide alone. It is safe to say that it's a matter of “not if but when” you'll face a hybrid PCB design. If you are already working in cutting-edge industries using hybrid construction, like 5G telecommunication, aerospace and defense, automotive electronics (EVs and autonomous vehicles), high-frequency RF and microwave communication, medical devices, and many more, then you've already overcome your fears and know the indulgence of trying the peanut butter cheeseburger.
With a combination of materials, the perfect fit for my analogy that’s very common in hybrid PCB designs is specialty materials, particularly for high-frequency and high-performance applications. Their unique properties make them ideal for demanding environments such as telecommunications, aerospace, and RF applications. A few advantages include a low and stable dielectric constant (Dk) that helps ensure faster signal propagation with minimal delay. Also, it has a low-loss tangent (or dissipation factor), which minimizes the signal energy loss as it travels through the board. Overall, it's an excellent material.
However, the disadvantages are that it’s a rather complex manufacturing process, especially when combined with a standard FR-4 PCB. It is directly related to the different thermal expansion of various materials. The stress during the fabrication process often causes cracks and delamination of the stackup.
Additionally, there is an inherent problem with its consistency making it challenging to work with. I often hear that it's like working with silly putty or, in my case, like a gooey peanut butter in the center of my hamburger. Manufacturers need to adapt their processes to accommodate the specific "consistency" of the specialty material they are working with, like how one might need to adjust a recipe based on the type of peanut butter used. Special care must be considered when working with a hybrid PCB design. Layering these materials requires careful alignment and pressure during the lamination process in PCB manufacturing. Too much pressure can lead to material deformation or damage, while too little pressure can result in poor adhesion between layers.
Then, when you think you've got it all correct and controlled, the material shifts on you, misaligning everything. That is an example of working with combined materials.
Despite these issues and challenges with a hybrid PCB design, it represents a cutting-edge solution in combining diverse materials to meet specific performance requirements. By leveraging the unique properties of each material, engineers can optimize signal integrity, thermal management, and mechanical stability, paving the way for advanced applications in telecommunications, aerospace, and consumer electronics.
When I'm hungry for a cheeseburger, I find myself seeking out peanut butter burgers wherever they may be. The same goes true for those hybrid PCB designs. Seek out these sorts of projects. The future is promising with hybrid designs.
John Watson is a professor at Palomar College, San Marcos, Calif.
More Columns from Elementary, Mr. Watson
Elementary Mr. Watson: The Road Ahead—Advances in PCB Design TechnologyElementary Mr. Watson: How to Reinvent Your Professional Journey
Elementary, Mr. Watson: Rules of Thumb—Guidelines vs. Principles for PCB Design
Elementary, Mr. Watson A Designer's Dilemma—Metric or Imperial Units?
Elementary, Mr. Watson: The Paradigm Shift of Silicon-to-System Design
Elementary, Mr. Watson: Debunking Misconceptions in PCB Design
Elementary, Mr. Watson: Mechatronics—The Swiss Army Knife of Engineering
Elementary, Mr. Watson: Cultivating a Culture of Collaboration