-
- News
- Books
Featured Books
- design007 Magazine
Latest Issues
Current IssueAll About That Route
Most designers favor manual routing, but today's interactive autorouters may be changing designers' minds by allowing users more direct control. In this issue, our expert contributors discuss a variety of manual and autorouting strategies.
Creating the Ideal Data Package
Why is it so difficult to create the ideal data package? Many of these simple errors can be alleviated by paying attention to detail—and knowing what issues to look out for. So, this month, our experts weigh in on the best practices for creating the ideal design data package for your design.
Designing Through the Noise
Our experts discuss the constantly evolving world of RF design, including the many tradeoffs, material considerations, and design tips and techniques that designers and design engineers need to know to succeed in this high-frequency realm.
- Articles
- Columns
- Links
- Media kit
||| MENU - design007 Magazine
Estimated reading time: 8 minutes
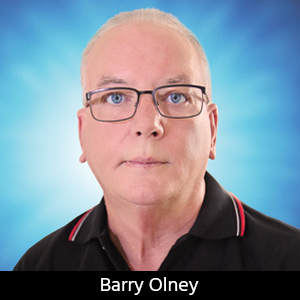
Beyond Design: Forget What You Were Taught
Ralph Morrison was a physicist who promoted the belief that electromagnetic energy flows in spaces, not the traces. That energy does not flow in the copper traces of a PCB, but rather the energy follows the traces acting as a waveguide and propagates through the dielectric material. This explains many electromagnetic (EM) effects such as radiation from outer microstrip layers and from stripline fringing fields, how components can be magnetically coupled, and why crosstalk is created by overlapping EM fields. But it also raises a few questions.
The simplistic approach to analyzing electronic circuits is to use the lumped element model. This methodology assumes that the attributes of the circuit—resistance, capacitance, and inductance—are concentrated into idealized electrical components connected by a network of perfectly conducting wires. However, that is not the case. As the frequency and rise time increase, these elements become distributed continuously through the substrate along the entire length of the trace. The copper trace and the adjacent dielectric materials become a transmission line, the skin effect forces current into the outer regions of the conductor, and frequency dependent losses impact the quality of the signal. The PCB trace now behaves as a distributed system with parasitic inductance and capacitance characterized by delay and scattered reflections. The behavior we are now concerned about, occurs in the frequency domain.
We were taught in circuit theory (and still are) that electric energy is made up of electrons moving in the conductors. Unfortunately, circuit theory does not consider the flight time of the signal, energy dissipation, and reflections which are key issues of digital logic design. It incorrectly teaches that:
- The conductors carry the energy
- The load instantly affects the flow of energy
- The transmission line has no losses
So now, it is time to forget what you were taught and instead think in terms of electromagnetic field theory. We must focus on the movement of EM fields and not on the flow of electrons.
The first law of thermodynamics states: Energy can neither be created nor be destroyed; it can only be transferred from one form to another. When an electromagnetic wave propagates from the source, it transfers energy to objects in its path. Electromagnetic fields transport all the energy on a circuit board. This carrier wave can transport information, or it can supply energy to power an IC. If information is carried, then the propagation of the wave alters the timing of the data and must be added to the delay of the circuit. The energy supplied to a load is carried in the space between the connecting conductors and not in the conductors themselves. This concept applies at all frequencies including DC. EM fields can also move energy in free space but not as DC.
Electromagnetic energy travels at the speed of light in a vacuum or air but is delayed by the dielectric materials used to construct multilayer PCBs. The relative permeability or dielectric constant (Dk) of the surrounding materials impacts the velocity of propagation (v) at the speed of light (c).
As the dielectric constant of a material increases, the velocity of propagation slows down. With a Dk = 4, the velocity is half the speed of light. If one uses different core and prepreg materials in the substrate, then each layer will have varying speeds depending on the dielectric constant. This will impact the timing of memory buses in particular.
What Happens at Low Frequency and DC?
Voltage differences can exist between points in space or between conducting surfaces. Electric fields exist at all frequencies including DC. In a field representation, lines of force start on a fixed amount of positive charge and terminate on the same amount of opposite charge. When the lines are close together, the forces are the greatest.
A small electric field within a conductor is required for current flow. When there is no current flow there is no field. The velocity of electrons in copper is extremely slow. In a typical geometry, the average velocity is less than 1 cm/s. This occurs because the electron density in a conductor is extremely high. What is important is the nature of the electric and magnetic fields.
At low frequencies (below 100 kHz), most of the field generated by a circuit returns to the source. This is a good example of where circuit theory can be put to good use. At DC, the fields are unchanging, and the energy is moving at about half the speed of light. We need to know the value of current flow to calculate voltage drop, power losses, and the magnetic field.
The fields associated with traces over a conducting plane are located under the traces. At frequencies below a kilohertz, the series inductive reactance between two points is lower than the resistance between these points. Therefore, at low frequencies current patterns are controlled by conductor resistance. The field required to move current in a solid plane at DC is very small.
Why Does a Thin Trace Overheat and Behave Like a Fuse?
The way I see it: electromagnetic energy moving through the dielectric induces current to flow in the conductors. The current is a result of the energy in the dielectric. It is an effect, not the cause. The trace and the plane bound the energy and thus it is the energy density between the conductors that determines the current density in the trace geometry. The wider and thicker the conductor (Figure 2), the more energy can be contained in the field. Thus, a larger cross-sectional area of copper is required to deliver higher energy at DC. However, the plane has sufficient capacity to support the energy flow. If the trace is too narrow, then the contained energy will dissipate as heat until the trace disconnects.
A DC power integrity simulator highlights areas of high current density, which will lead to higher temperatures. A low thermal resistance ensures that the heat is transferred through the material much faster. This resistance is directly proportional to the length of the thermal path and inversely proportional to the cross-sectional area and thermal conductivity of the thermal path.
For instance, if the load is shorted, then the EM field will try to maintain the output voltage by providing more energy. As the energy level of the field increases, it looks for the weakest link, which is the point of lowest resistance on the trace segment. The excess energy will then transform into thermal energy, vaporizing the trace at the weakest point.
The trace also cools by conduction through the substrate. But as the trace temperature increases, the dielectric material beneath degrades, passing through the stages of the glass transition to thermal decomposition and finally delamination. Deterioration of the underlying dielectric material will reduce the effective cooling capacity of the material and thus increase the temperature of the trace.
If there is current flow in a conductor, an electric field must exist. At DC, this field will be the same at any depth. However, a changing magnetic field associated with AC flow forces the current to bind to the perimeter of the conductors. This is known as the skin effect and applies to digital circuits, where currents flow very near the surface of the conductors. For a sine wave current at 1 MHz, the skin depth in copper is only 66 µm. This means that the current at this depth is the surface current reduced by 8.68 dB. At 1 GHz, the skin depth is 0.066 µm and decreases with higher frequency.
As frequencies and rise times continue to increase, the PCB designer needs to consider the path of electromagnetic fields through the substrate. To fully understand the operation of digital circuits, we must focus on the movement of EM fields and not on the flow of electrons. This also applies to DC analysis of the power distribution network. Rather than considering the current capacity of a conductor, one should allow for the amount of field energy that the conductor can confine.
Key Points
- Energy does not flow in the copper traces of a PCB, but rather the energy follows the traces acting as a waveguide and propagates through the dielectric material.
- The lumped element model assumes that the attributes of the circuit—resistance, capacitance, and inductance—are concentrated into idealized electrical components connected by a network of perfectly conducting wires.
- A distributed system disperses elements through the substrate along the entire length of the transmission line and is characterized by delay and scattered reflections.
- Forget what you were taught in circuit theory and instead think in terms of electromagnetic field theory. We must focus on the movement of EM fields and not on the flow of electrons.
- An EM carrier wave can transport information, or it can supply energy to power an IC.
- When information is carried, the propagation of the wave alters the timing of the data and must be added to the delay of the circuit.
- Electric fields exist at all frequencies including DC.
- The velocity of electrons in copper is extremely slow. In a typical geometry, the average velocity is less than 1 cm/s.
- The fields associated with traces over a conducting plane are located under the traces.
- At frequencies below a kilohertz, the series inductive reactance between two points is lower than the resistance. Therefore, at low frequencies, current patterns are controlled by conductor resistance.
- Electromagnetic energy moving through the dielectric induces current to flow in the conductors.
- The trace and the plane bound the energy and thus it is the energy density between the conductors that determines the current density in the trace geometry.
- The wider and thicker the conductor, the more energy can be contained in the field.
- If the trace is too narrow, then the contained energy will dissipate as heat.
- A DC power integrity simulator highlights areas of high current density, which will lead to higher temperatures.
- The trace also cools by conduction through the substrate.
- The skin effect applies to digital circuits, where currents flow very near the surface of the conductors.
Resources
- Beyond Design by Barry Olney: The Wavelength of Electromagnetic Energy, The Big Bang-Lumped Element to Distributed System, Surface Roughness.
- An interview with Rick Hartley discussing Ralph Morrison by Judy Warner.
- “Fast Circuit Boards” by Ralph Morrison
- The Energy Density of Electromagnetic Waves” by Cadence.
This column originally appeared in the November 2022 issue of Design007 Magazine.
More Columns from Beyond Design
Beyond Design: The Metamorphosis of the PCB RouterBeyond Design: Radiation and Interference Coupling
Beyond Design: Key SI Considerations for High-speed PCB Design
Beyond Design: Electro-optical Circuit Boards
Beyond Design: AI-driven Inverse Stackup Optimization
Beyond Design: High-speed Rules of Thumb
Beyond Design: Integrated Circuit to PCB Integration
Beyond Design: Does Current Deliver the Energy in a Circuit?