-
- News
- Books
Featured Books
- design007 Magazine
Latest Issues
Current IssueAdvanced Packaging and Stackup Design
This month, our expert contributors discuss the impact of advanced packaging on stackup design—from SI and DFM challenges through the variety of material tradeoffs that designers must contend with in HDI and UHDI.
Rules of Thumb
This month, we delve into rules of thumb—which ones work, which ones should be avoided. Rules of thumb are everywhere, but there may be hundreds of rules of thumb for PCB design. How do we separate the wheat from the chaff, so to speak?
Partial HDI
Our expert contributors provide a complete, detailed view of partial HDI this month. Most experienced PCB designers can start using this approach right away, but you need to know these tips, tricks and techniques first.
- Articles
- Columns
Search Console
- Links
- Media kit
||| MENU - design007 Magazine
Estimated reading time: 8 minutes
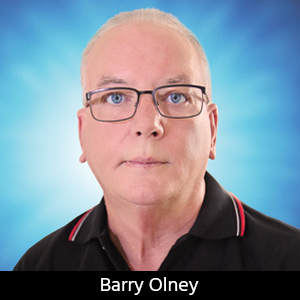
Beyond Design: The Interaction of Electromagnetic Fields
When two (or more) electromagnetic fields overlap or meet, they add vectorially at each point in space. Fields have direction and polarity. At any point in space, there can be only one field, so at some spatial points, they will cancel each other, and at others, they will re-enforce each other. James Clerk Maxwell described electromagnetic fields as being linear. Linearity implies superposition, meaning that the fields do not merge with each other but rather add vectorially—distorting the signal. This applies to both static (DC) and time-varying (AC) fields.
When an electromagnetic field propagates along a transmission line, there are electric field lines between the signal and return paths and rings of magnetic field lines around the signal and return path conductors. These fields are not confined to the immediate space between the signal and return paths but rather, fringe fields spread out into the surrounding volume.
In a stripline (inner) configuration (Figure 1a), the electromagnetic field propagates between the planes, and the return displacement current flows in close proximity (mainly) to the nearest plane. As the frequency increases, the current is forced into the outer surface of the copper, due to the skin effect, dramatically increasing loss. Figure 1b shows the electromagnetic field in a microstrip (outer layer) configuration. Electric fields terminate when they come into contact with a solid plane, whereas magnetic fields tend to radiate into the dielectric or air above or below the PCB. However, stripline (embedded signals) are rather confined by the adjacent planes and only emit radiation on the fringing fields of the PCB edge. This is a good reason to embed all critical signals.
Both electric and magnetic field coupling can occur on PCBs; however, crosstalk usually comes from electric field-based capacitive coupling. Trace segments running in parallel on the same layer or running vertically (broadside) between two layers are extremely susceptible to crosstalk. Parallel traces act as capacitor plates separated by a dielectric. If there is a change in voltage, it will alter the electric field and generate a displacement current leading to crosstalk. Mutual capacitance between the two lines couples energy in both directions on the victim trace (Figure 2a).
Inductive coupling depends on the magnitude of the magnetic field surrounding the victim trace. The magnetic field change generated by the aggressor signal surrounding the victim trace results in a changing conduction current. Mutual inductance between the lines couples energy onto the victim trace segment in the backward direction (Figure 2b)
Though both mutual capacitance and mutual inductance play a role in crosstalk, there are two regimes to consider. When the return path is a wide, uniform plane, as is the case for most coupled transmission lines in a multilayer PCB, the capacitively coupled energy and inductively coupled energy are of the same order of magnitude. However, when the return path is a single lead, in a package, or a single pin in a connector, the inductively coupled energy is much larger than the capacitively coupled energy. In this case, the noise behavior is dominated by the inductively coupled energy. The noise on the victim line is driven by a dI/dt in the aggressor net, which happens at the rising and falling edges of the signal when the driver switches.
Electromagnetic fields have direction and polarity. Forward (or far-end crosstalk, FEXT) and backward (or near-end crosstalk, NEXT) refer to the direction the crosstalk travels along a victim transmission line. Forward crosstalk propagates in the same direction as the aggressor signal, while backward crosstalk propagates in the opposite direction.
- FEXT = (Capacitive coupling - Inductive coupling)/2
- NEXT = (Capacitive coupling + Inductive coupling)/4
Figure 3: Forward (FEXT) and backward (NEXT) crosstalk for microstrip and stripline configurations.
In an outer layer microstrip configuration, the mutual capacitive coupling between adjacent traces is generally weaker than the mutually inductive coupling, driving the FEXT co-efficient negative (Figure 3a). However, forward crosstalk does not exist in the stripline configuration. The fine balance between capacitive and inductive coupled crosstalk produces no observable forward crosstalk (Figure 3b). And, the NEXT component is halved. So, all other factors being equal, here is just another good reason why one should always route critical high-speed signals on the inner layers of a multilayer PCB.
Figure 4 illustrates the “backward crosstalk critical length.” This is a measure of how far an aggressor line and a victim line can route in parallel before the amplitude of backward crosstalk (NEXT) reaches its maximum level. Parallelism beyond this length will widen the coupled pulse in the victim line, but will not increase its amplitude. As rise time gets faster, ”critical length” becomes shorter. In today’s systems, with extremely fast IC output switching times, almost any parallelism between lines can be a problem. As seen in the graph of Figure 4, with a rise time of 200 ps, backward crosstalk reaches its maximum amplitude in 12.5 mm (1/2 in) of parallelism between lines. The absolute amplitude is determined by the distance between the aggressor and victim lines relative to their height above the reference plane (ground plane). For this reason, it is extremely important to route all traces on a layer that is one dielectric distance above or below a ground plane and to keep the dielectric thickness as thin as reasonably possible, within the constraints of good manufacturability rules.
Having a wide uniform plane as the return path is the configuration for the lowest radiation and crosstalk. Anything that changes the return path from a wide uniform plane will increase the amount of coupled noise between transmission lines. Usually this happens when the signal goes through a connector, and the return paths for more than one signal path are now shared by one of the pins rather than by a plane—the inductively coupled noise increases much more than the capacitively coupled noise. Simultaneous switching noise occurs mostly in connectors, IC packages, and vias, where the return path conductor is not a wide, uniform plane. Ground bounce is really a special case of crosstalk caused when the fields from several lines overlap and couple into one another. As the fields all attempt to couple to a single reference line in the connector or IC package, or into a single ground via, the return currents overlap, creating very high mutual inductance.
Engineers often employ guard traces to help lower crosstalk. In a well-designed PCB with the ideal number of ground planes, guard traces offer little, if any, value unless properly attached to the ground plane(s) of the board. An ungrounded guard trace (Figure 5a) or one attached to the ground plane(s) at one or both ends only (middle) can create a bandpass filter—an LC network—that radiates at one-quarter wavelength of its resonant frequency. Whereas, a guard trace that is grounded every 1/12th wavelength (bottom) prevents radiation and drastically lowers cross-coupling.
On one- and two-layer PCBs, with no ground planes, even guard traces that are connected to the 0V reference points at both ends only, are often better than no return reference. That said, employing one- and two-layer PCBs without planes is a very poor design practice. The traces have an impedance of 376 ohms (impedance of free space) and will often cause more problems than they solve.
To minimize the detrimental interaction of electromagnetic fields, it is good design practice to:
- Separate asynchronous signals, unrelated signals, and signals of different potentials.
- Avoid long parallel trace segments to minimize their coupling.
- Route all critical signals embedded between the planes to reduce crosstalk.
- Keep other signals away from I/O signals and connectors. Never allow anything to route into the I/O area of the board, except I/O lines.
- Use low dielectric constant material and closely couple the signal trace to the reference plane to decrease the radiation.
Key Points
- EM fields do not merge with each other but rather add vectorially—distorting the signal.
- Crosstalk usually comes from electric field-based capacitive coupling of trace segments running in parallel.
- Mutual capacitance between the two lines couples energy in both directions on the victim trace.
- A wide uniform plane as the return path is the configuration for the lowest radiation and crosstalk.
- Mutual inductance between the lines couples energy onto the victim trace segment in the backward direction.
- When multiple fields couple to a single reference line, in a connector or IC package, or into a single ground via, the return currents overlap, creating very high mutual inductance.
- Electromagnetic fields have direction and polarity.
- Forward crosstalk does not exist in the stripline configuration due to the fine balance between the capacitive and inductive coupling.
- For a rise time of 200 ps, backward crosstalk reaches its maximum amplitude in 12 mm of parallelism between lines.
- The amplitude of crosstalk is determined by the distance between the aggressor and victim lines relative to their height above the reference plane. It is extremely important to route all traces on a layer that is one dielectric distance above or below a ground plane.
- A guard trace that is grounded every 1/12th wavelength (bottom) prevents radiation and drastically lowers cross-coupling.
Resources
- Beyond Design columns by Barry Olney: “Return Path Discontinuities,” “The Dark Side—Return of the Signal,” “Stackup Configurations to Mitigate Crosstalk.”
- “Noise, EMI & SI and Single-ended Crosstalk,” by Rick Hartley.
- “Signal and Power Integrity Simplified,” by Eric Bogatin.
Rick Hartley is the principal engineer at R Hartley Enterprises and has been in the industry for over 50 years. He is one of the primary consultants for PCB manufacturing and design companies. Rick has also conducted classes worldwide on EMI, signal integrity, and various other electrical topics for the last 30 years.
This column originally appeared in the March 2023 issue of Design007 Magazine.
More Columns from Beyond Design
Beyond Design: High-speed Rules of ThumbBeyond Design: Integrated Circuit to PCB Integration
Beyond Design: Does Current Deliver the Energy in a Circuit?
Beyond Design: Termination Planning
Beyond Design: Dielectric Material Selection Guide
Beyond Design: The Art of Presenting PCB Design Courses
Beyond Design: Embedded Capacitance Material
Beyond Design: Return Path Optimization