-
-
News
News Highlights
- Books
Featured Books
- design007 Magazine
Latest Issues
Current IssueAll About That Route
Most designers favor manual routing, but today's interactive autorouters may be changing designers' minds by allowing users more direct control. In this issue, our expert contributors discuss a variety of manual and autorouting strategies.
Creating the Ideal Data Package
Why is it so difficult to create the ideal data package? Many of these simple errors can be alleviated by paying attention to detail—and knowing what issues to look out for. So, this month, our experts weigh in on the best practices for creating the ideal design data package for your design.
Designing Through the Noise
Our experts discuss the constantly evolving world of RF design, including the many tradeoffs, material considerations, and design tips and techniques that designers and design engineers need to know to succeed in this high-frequency realm.
- Articles
- Columns
- Links
- Media kit
||| MENU - design007 Magazine
Estimated reading time: 6 minutes
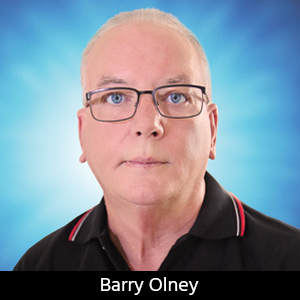
Beyond Design: Radiation and Interference Coupling
Radiation and interference coupling pose significant challenges to the performance and reliability of high-speed digital designs. Whether it's the unintended emission of electromagnetic waves or the interaction of signals between adjacent circuits, these phenomena can lead to unwanted noise, signal distortion, and even system malfunctions. Understanding the mechanisms behind radiation and interference coupling is crucial for electronics designers seeking to design robust and efficient systems.
The characteristic impedance of a transmission line is determined by its conductor geometry, and it represents the ratio of the electric field (E) intensity to the magnetic field (H) intensity as they propagate along the line. On PCBs, the proximity of traces to each other and to conducting planes restricts the amount of field energy that radiates from any individual transmission line. For traces situated between conducting planes in a stripline configuration, board radiation is almost nonexistent (with the exception of edge fringing fields). However, on a typical outer (microstrip) layer, where hundreds of transmission lines may be active simultaneously, even a small portion of energy escaping from each trace can collectively produce a significant radiated field.
In Figure 1, electric field lines are plotted in blue. These can be thought of as the "lines of force." Note that they begin and end on conductor surfaces (where physical charges reside). They refract (change direction) at boundaries between different dielectrics. Electric fields, which capacitively couple signals into a nearby trace, are absorbed by the plane but still tend to radiate noise outward.
Magnetic fields are plotted in red. These existed in circular form around the trace along which the electric potential (voltage) is a constant. They form closed contours around one or more conductors. The inductance of the trace depends on the geometry of the circuit and the magnetic properties of the media containing the field. Electromagnetic fields are totally blocked by a solid plane.
The differential pair in Figure 2 illustrates the electromagnetic field coupling between the two trace segments. Magnetic fields tend to radiate into air, which is actually just another dielectric with a dielectric constant of 1.
The energy carried along a transmission line travels within the electromagnetic field between the conductors. Radiation or field coupling occurs only in areas where the wave amplitude is changing. This happens at the leading edge as it moves down the line. When the rise time increases, the radiating area grows larger.
For example, during a rise time of 100 ps, a wave can travel 1.5 cm. If the transmission line is series-terminated at the logic source and measures 6 cm in length, the radiation pattern persists for a full round trip of the wave, approximately 800 ps. The radiating pattern is identical for both the outgoing and reflected waves. For fast leading edges, the radiation observed from a distance appears to come from a moving current element, effectively behaving like a moving dipole.
Radiation and cross-coupling happen wherever there are changes in field patterns. For outer traces (microstrip), most of the electromagnetic field is contained within the dielectric material, while a smaller portion is in the air. The part of the field in the air travels more quickly, which causes the leading edge of the signal to spread out. This stretching of the signal impacts the characteristics of the crosstalk.
Crosstalk is caused by capacitive and inductive coupling (Figure 3). Capacitive coupling causes signal voltages to couple into nearby nets, also referred to as forward or far-end crosstalk (FEXT). Capacitive coupling can lead to unintended interference in the affected conductor, typically observed as noise or signal distortion. High-frequency signals are particularly susceptible to capacitive coupling due to the stronger and more dynamic electric fields they generate.
Inductive coupling also causes signal currents to couple into nearby nets, a process referred to as backward or near-end crosstalk (NEXT). The magnetic component of cross-coupling can distort signals, especially in high-speed circuits where rapid changes in current produce stronger and more dynamic magnetic fields. These distortions can manifest as noise or unintended signal deviations in nearby conductors.
Factors Influencing Capacitive Coupling
- Proximity: The closer the conductors are, the higher the capacitance between them, leading to stronger coupling.
- Voltage levels: Higher voltages on the source conductor create stronger electric fields, increasing coupling.
Factors Influencing Magnetic Coupling
- Proximity of conductors: The closer the conductors are to each other, the stronger the magnetic coupling due to increased mutual inductance.
- Current variations: Rapid changes in current generate dynamic magnetic fields that can intensify coupling effects.
- Orientation and geometry: The layout and relative orientation of the conductors impact the direction and strength of the magnetic field, influencing how coupling occurs.
- Frequency: Higher frequency signals tend to generate stronger magnetic fields, increasing the likelihood and intensity of coupling.
Mitigation Techniques
- Spacing: Increasing the distance between conductors. Alternatively, if real estate is of concern, decrease the trace-to-plane spacing.
- Shielding: Placing a grounded shield between conductors can block electric fields and reduce coupling.
- Signal voltage: Reducing the voltage levels of the aggressor signals can weaken electric fields and decrease coupling.
- Dielectric material: Increasing the dielectric constant slows down the signal propagation, reducing interaction and improving the containment of the field.
Energy can also radiate from the board edges. The energy stored between the conducting planes works with the energy stored in the decoupling capacitors. When energy is needed, electromagnetic waves travel through the decoupling capacitors and the cavity between the planes. Reflections of these waves send energy back to the point where it’s required. However, when a wave reaches the edge of the board, the open circuit causes the wave to reflect, doubling its voltage. Different frequency wavefronts reach the board edge at different times, and each reflection doubles the wave's amplitude.
There are various approaches to reducing radiation edge effects from the PCB. In many cases, energy can be reflected, possibly creating additional internal cavity resonance effects and coupling to internal vias, also resulting in increased radiation. However, termination along the board edges reduces the resonance peaks. In practice, this means approximating a continuous structure with resistors, spaced around the perimeter, that are AC coupled with a ceramic capacitor of sufficient capacitance to allow the resulting impedance to appear predominately resistive at and above the lowest frequency of interest.
Effective mitigation techniques, including proper spacing, shielding, and strategic termination along board edges, are essential for maintaining signal integrity and minimizing radiated emissions. By addressing these challenges, engineers can design PCBs that perform reliably even in demanding electromagnetic environments.
Key Points
- The characteristic impedance represents the ratio of the electric field intensity to the magnetic field intensity as they propagate along the line.
- There is negligible radiation for traces situated between conducting planes in a stripline configuration.
- On a typical outer (microstrip) layer, even a small portion of energy escaping from each trace can collectively produce a significant radiated field.
- Electromagnetic fields are totally blocked by a solid plane.
- Radiation or field coupling occurs only in areas where the wave amplitude is changing. This happens at the leading edge as it moves down the line. When the rise time increases, the radiating area grows larger.
- Crosstalk is caused by capacitive and inductive coupling.
- Energy can radiate from the board edges. The energy stored between the conducting planes works together with the energy stored in the decoupling capacitors.
- When a planar wave reaches the edge of the board, the open circuit causes the wave to reflect, doubling its voltage.
- Effective mitigation techniques, including proper spacing, shielding, and strategic termination along board edges, are essential.
Resources
- Beyond Design columns by Barry Olney: “Electromagnetic Fields Parts 1 & 2,” “Fringing Fields,” “Dampening Plane Resonance With Termination”
- “Fast Circuit Boards” by Ralph Morrison
This column originally appeared in the May 2025 issue of Design007 Magazine.
More Columns from Beyond Design
Beyond Design: The Metamorphosis of the PCB RouterBeyond Design: Key SI Considerations for High-speed PCB Design
Beyond Design: Electro-optical Circuit Boards
Beyond Design: AI-driven Inverse Stackup Optimization
Beyond Design: High-speed Rules of Thumb
Beyond Design: Integrated Circuit to PCB Integration
Beyond Design: Does Current Deliver the Energy in a Circuit?
Beyond Design: Termination Planning