-
-
News
News Highlights
- Books
Featured Books
- design007 Magazine
Latest Issues
Current IssueAll About That Route
Most designers favor manual routing, but today's interactive autorouters may be changing designers' minds by allowing users more direct control. In this issue, our expert contributors discuss a variety of manual and autorouting strategies.
Creating the Ideal Data Package
Why is it so difficult to create the ideal data package? Many of these simple errors can be alleviated by paying attention to detail—and knowing what issues to look out for. So, this month, our experts weigh in on the best practices for creating the ideal design data package for your design.
Designing Through the Noise
Our experts discuss the constantly evolving world of RF design, including the many tradeoffs, material considerations, and design tips and techniques that designers and design engineers need to know to succeed in this high-frequency realm.
- Articles
- Columns
- Links
- Media kit
||| MENU - design007 Magazine
Estimated reading time: 4 minutes
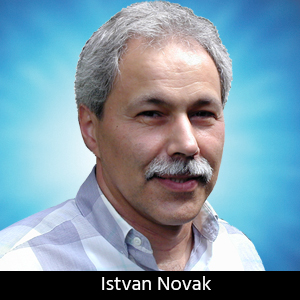
PDN Measurements: Reducing Cable-Braid Loop Error
There are several reasons why PDN validation is more convenient in the frequency domain rather than in the time domain. We may cover those reasons later in a separate column.
When it comes to simulating or measuring PDN components, modules or systems, we usually want to do it as a function of frequency. At low and mid frequencies, where the self-impedance of a device under test (DUT) may reach milliohm values or even less, a fundamental challenge in measurement is the connection to the DUT. Unless we measure a single component in a well-constructed fixture, the homemade connections from the instrument to the DUT will introduce too much error.
The top scheme in Figure 1 shows a vector network analyzer (VNA) connecting to the DUT with a cable. Our DUTs (for instance, a DC-DC converter or a populated board) typically do not have connectors and therefore we need to have a short piece of wire (possibly the center wire of the coax cable or connector) to attach to the DUT. However, just 40 milli-inches (1 millimeter) of wire outside of the calibration loop may result in an error as much as 1 milliohm resistance and 1 nH inductance. The solution is to use the AC equivalent of the DC four-wire Kelvin measurement approach, shown on the bottom scheme of Figure 1.
Figure 1: Illustration of connection error with a single connection to the DUT (on the top), and block schematics of the Two-port Shunt-through measurement (on the bottom).
The two-port shunt-through connection works well for very small impedances. The DUT impedance can be resolved from the measured S21 parameter as follows:
where ZDUT is the unknown complex impedance to be measured, ZVNA is the reference impedance of VNA (usually 50 ohm) and S21 is the measured complex response by the VNA.
The two-port shunt-through connection is a good fit for vector network analyzers or frequency response analyzers, which inherently have at least two ports, one being the source at any given time. However, by using two connections attached to the same DUT, we have just created a new problem: Unless one or both ports are floating, the reference connections going to the two ports form a ground loop and we end up with a significant error floor. This is explained in Figure 2. We assume very low frequencies (or DC), so that we need to consider only resistance and ignore inductance. The test current flowing through the DUT (for sake of simplicity, it is represented by a short) creates a Ve voltage drop across the parallel equivalent of the two cable-braid resistances: Rb1 x Rb2. Therefore, instead of the expected zero value, the reading at Port 2 will be this Ve voltage, which represents the Rb1 x Rb2 resistance.
Figure 2: Illustration of cable-braid loop error.
This cable-braid error floor gradually diminishes as the inductive reactance of the cable opens up the loop. By the time we get up into the MHz frequency range, this error usually diminishes. At low frequencies, until recently we had to either use an external floating-input differential amplifier or artificially increase the common-mode inductance of the cable by adding ferrite beads or threading the cable through a ferrite toroid.
The Agilent E5061B LF-RF network analyzer takes a different approach by using semi-floating references for its gain-phase port inputs [1]. The equivalent low-frequency impedance is approximately 30 ohms and this reduces the cable-braid loop's error dramatically. The concept is illustrated in Figure 3, and its results are shown in Figure 4.
Assuming that the ZDUT impedance is much lower than 50 ohms, the Va error voltage, created by the test current flowing through the cable-braid impedances, is similar in magnitude to Ve in Figure 2. However, the received VT voltage will contain only a very tiny attenuated portion of this error voltage, Vb2.
Figure 3: Concept of semi-floating ground in the gain-phase test port of E5061B. Courtesy of Agilent Technologies.
From the Va = Vg + Vb2 vector triangle we can calculate the actual Vb2 error voltage as
Since Zg2 >> Zb2, the voltage at the T input will be very close to the correct value: VT ~ Vo. For instance, assuming 30 milliohm braid resistance, the 30 ohm semi-floating ground impedance will reduce this error to 30 microOhm.
Figure 4: Illustration of effectiveness of semi-floating ground. Courtesy of Agilent Technologies.
Figure 4 was measured using a homemade reference piece that has one milliohm DC resistance with some series inductance. The left four traces are measurement results with a conventional grounded receiver. Here, (a) represents the measurement result without attaching anything to the cables, which indicates the measurement error due to the cable braid loop in the low-frequency range. The other three traces (b) through (d) show the measurement results by adding an increasing number of ferrite cores to the test cable. The error is reduced by adding inductance, but the result is still not perfect. On the other hand, the right plot shows the low-frequency reading with the semi-floating ground in the E5061B VNA, which is flat at the correct value.
The E5061B VNA can accurately measure milliohm impedances. More details can be found in [2] about PDN measurements and in [3] about the measurements with E5061B.
References
[1] http://cp.literature.agilent.com/litweb/pdf/5990-4391EN.pdf
[2] Frequency-Domain Characterization of Power Distribution Networks, Artech House, 2007.
[3] Accuracy Improvements of PDN Impedance Measurements in the Low to Middle Frequency Range, DesignCon 2010, February 1-4, 2010, Santa Clara, CA. Available online at www.electrical-integrity.com
About the author
Istvan Novak is a distinguished engineer at SUN Microsystems working on signal and power integrity designs of mid-range servers and new technology developments. He can be reached at istvan.novak@att.net.
More Columns from Quiet Power
Quiet Power: An Evolution in PCB Design CostsQuiet Power: The Effect on SI and PI Board Performance
Quiet Power: 3D Effects in Power Distribution Networks
Quiet Power: Noise Mitigation in Power Planes
Quiet Power: Uncompensated DC Drop in Power Distribution Networks
Quiet Power: Ask the Experts—PDN Filters
Quiet Power: Friends and Enemies in Power Distribution
Quiet Power: Be Aware of Default Values in Circuit Simulators