-
-
News
News Highlights
- Books
Featured Books
- design007 Magazine
Latest Issues
Current IssueCreating the Ideal Data Package
Why is it so difficult to create the ideal data package? Many of these simple errors can be alleviated by paying attention to detail—and knowing what issues to look out for. So, this month, our experts weigh in on the best practices for creating the ideal design data package for your design.
Designing Through the Noise
Our experts discuss the constantly evolving world of RF design, including the many tradeoffs, material considerations, and design tips and techniques that designers and design engineers need to know to succeed in this high-frequency realm.
Learning to Speak ‘Fab’
Our expert contributors clear up many of the miscommunication problems between PCB designers and their fab and assembly stakeholders. As you will see, a little extra planning early in the design cycle can go a long way toward maintaining open lines of communication with the fab and assembly folks.
- Articles
- Columns
Search Console
- Links
- Media kit
||| MENU - design007 Magazine
Estimated reading time: 6 minutes
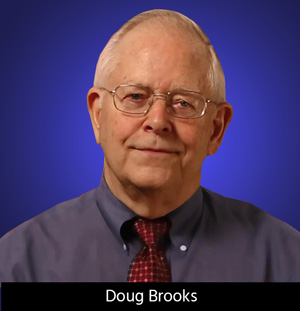
Contact Columnist Form
Propagation Speed in Microstrip: Slower Than We Think
Most of us are aware of the rule-of-thumb that PCB signals travel at 6”/nsec. And we have at least a vague idea of where that comes from. Well, here are the facts.
The Speed of Light
Light travels at about 186,282 miles/sec (see Note 1). Multiply that by 5,280 feet/mile and 12 inches/foot and you get almost exactly 11.8”/nsec. Light slows down in any other medium. It slows down by the square root of the relative dielectric coefficient of the medium, or
Since most of our boards are made from FR-4, and since the relative dielectric coefficient of FR-4 is approximately 4, and since the square root of 4 is 2, then it follows from Equation 1 that the propagation speed of signals on our boards is about 11.8”/2 = 5.9”/nsec, which is just about 6”/nsec.
Before we look at this more closely, here is an illustration I use sometimes in my classes. Figure 1 is a scene I photographed near Redmond, Oregon, just north of Bend. I have added two pairs of “poles” to the picture and strung a copper wire between each pair. The copper wires are identical in length, but one drops down into the lake while the other extends out beyond it. The question is this: If I send a signal down each wire at identical times, will the signals (a) reach the opposite ends at the same time, (b) arrive faster at the end of the wire that does not dip into the water, or (c) arrive faster at the end of the wire that does drop into the water? (See Note 2.)
Figure 1. Relative propagation speed of signals.
The correct answer is (b). Here’s why. When a signal propagates down a wire or trace, by definition, the current on the trace changes. Changes in current are accompanied by a change in the electromagnetic field around the wire. The current cannot get out in front of the electromagnetic field, and the electromagnetic field can’t get out in front of the current. The propagation speed is not determined by how fast the current can travel down the wire; it is determined by how fast the electromagnetic field can propagate in the medium it is in. (Go back and read that sentence one more time! It is the heart of this article.) The propagation speed of the electromagnetic field in the water is much slower than it is in air (approximately 9 times slower), so the propagation speed is faster for the wire that does not dip into the water.
Equation 1 is very precise on PCBs under the right conditions. Those conditions are:
- The dielectric material surrounding the trace is homogeneous (uniform), and
- We actually know the relative dielectric coefficient for the material.
These conditions are approximately met in a stripline environment. The first assumption is often pretty good for stripline. The second assumption can be problematic. But if we DO know the relative dielectric coefficient of the material with precision, then we can use Equation 1 to determine the signal propagation speed very precisely. Note, in particular, that in Stripline environments, 100% of the electromagnetic field is contained within the dielectric material.
Microstrip
The propagation speed of a microstrip trace, however, is much more problematic. Unlike the stripline environment, the electromagnetic field in a microstrip environment is partly within the dielectric material and partly in air (and partly, perhaps in solder coat and conformal coating). Consider Figure 2. It shows the electric field distribution for three different microstrip traces (the magnetic fields are omitted for clarity.)
Figure 2. Electric field distribution in microstrip traces.
Let the trace shown in the top left part of the figure be a typical trace. Note that if we move that trace closer to the plane (top right in the figure), more of the electric field is contained within the dielectric material. Or, as shown in the bottom of the figure, if the trace is very wide, again more of the electric field is within the dielectric material.
We can therefore make these generalizations:
- For very narrow microstrip traces placed relatively far above the underlying plane, the highest percentage of the electromagnetic field will be in the air above the dielectric material. These traces will have the fastest microstrip propagation speeds.
- For very wide microstrip traces placed relatively close to the underlying plane, the highest percentage of the electromagnetic field will be in the dielectric material between the trace and the plane. These traces will have the slowest propagation speeds, (in the limit) approaching those of stripline traces surrounded by the same dielectric.
Propagation speeds in microstrip slow down as traces get closer to the plane or as trace widths widen (all other things equal.)
Estimating Microstrip Propagation Speeds and Times
A rule of thumb for estimating microstrip propagation speeds was proposed some time ago – you may be aware of it. It suggested that we substitute a modified value of er into Equation 1. That modified value was given as:
The most obvious problem with this approach is that it is a constant, and does not take into consideration either the trace width or the height above the underlying plane. Based on a study I did a few years ago I have come up with (what I believe is) a more accurate prediction of the propagation time (not speed, which is the inverse of the propagation time). It is based on the idea that the propagation time in microstrip is the same as the propagation time in stripline, adjusted by a factor, or Equation 3 (see Note 3):
The fraction is given by Equation 4:
Admittedly, this looks pretty intimidating. And even it doesn’t take into consideration other complexities such as solder coat and conformal coating. Some high-end design systems will do these adjustments for you (see Note 4.) But if the determination of or the designing in of highly precise propagation times is important in your application, consider keeping all the traces in a stripline environment. Propagation times are much more controllable there.
Notes:
- Light travels at this speed in a vacuum. In air, it travels at a slightly slower speed, about one part in 10,000. From a practical standpoint, for those of us on Earth, the difference is negligible.
- I had a student once declare “This is a trick question! Everyone knows the water will short out the signal and it won’t get to the far end at all!” While I am not above trick questions, this is not one of those times.
- For a full explanation of how this formula was derived, see: “Microstrip Propagation Times; Slower Than We Think” Available at www.ultracad.com.
- We at UltraCAD use the Mentor Graphics Expedition design system and it will do this adjustment. I don’t know about other design systems.
Douglas Brooks has an MS/EE from Stanford University and a Ph.D. from the University of Washington. He has spent most of his career in the electronics industry in positions of engineering, marketing, general management, and as CEO of several companies. He has owned UltraCAD Design Inc. since 1992. He is the author of numerous articles in several disciplines, and has written articles and given seminars all over the world on Signal Integrity issues since founding UltraCAD. His book, Printed Circuit Board Design and Signal Integrity Issues was published by Prentice Hall in 2003. Visit his Web site at www.ultracad.com.
More Columns from Brooks' Bits
Brooks' Bits: Internal Trace Temperatures—More Complicated Than You ThinkBrooks' Bits: Electromagnetic Fields, Part 3 - How They Impact Coupling
Brooks' Bits: Electromagnetic Fields, Part 2: How They Impact Propagation Speed
Brooks' Bits: How Electromagnetic Fields Determine Impedance, Part 1
Trace Currents and Temperature, Part 4: Via Heat
Trace Currents and Temperature, Part 3: Fusing Currents
Trace Currents and Temperature, Part 1: The Basic Model
The Skinny on Skin Effect, Part 3: Crossover Frequency