-
- News
- Books
Featured Books
- design007 Magazine
Latest Issues
Current IssueThe Designer of the Future
Our expert contributors peer into their crystal balls and offer their thoughts on the designers and design engineers of tomorrow, and what their jobs will look like.
Advanced Packaging and Stackup Design
This month, our expert contributors discuss the impact of advanced packaging on stackup design—from SI and DFM challenges through the variety of material tradeoffs that designers must contend with in HDI and UHDI.
Rules of Thumb
This month, we delve into rules of thumb—which ones work, which ones should be avoided. Rules of thumb are everywhere, but there may be hundreds of rules of thumb for PCB design. How do we separate the wheat from the chaff, so to speak?
- Articles
- Columns
Search Console
- Links
- Media kit
||| MENU - design007 Magazine
Estimated reading time: 3 minutes
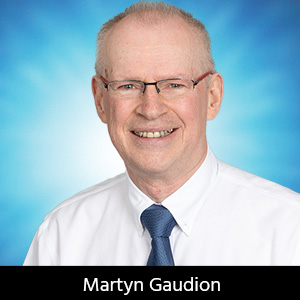
Transmission Lines – a Voyage From Dc – No, Not Washington ...Part 2
In the second part of this two-part article we continue on our voyage through a transmission line from DC onwards and upwards through the frequency spectrum, step by step exploring the characteristics from very low to ultra high frequencies. In Part 1 we charted the impedance from infinity at DC to the GHz region where it reaches the steady state value of its characteristic impedance, in this case, 50 Ohms. In Part 2 we look at mechanisms that cause loss in the line, robbing the signal of its energy as it transits the line.
The following graph, Figure 4, shows the impedance magnitude flattening out at 50 Ohms in the range of 1GHz through 20GHz.
So that takes us from DC to the GHz region, and we have seen so far that the characteristic impedance falls from infinity at DC to a steady state of 50 Ohms and flattens out.
Surely something else happens at higher frequencies? Everyone asks about line performance as data rates loft into the multi GHz region. Whilst the instantaneous impedance stays roughly constant, the line can no longer be treated as lossless as a range of loss mechanisms come into play to rob the signal of its energy as it transits the line.
Skin depth
Skin depth is the first loss mechanism that must be taken into account; as the frequency is increased currents increasingly flow on a thinner layer closer and closer to the surface of the trace; this reduces the effective cross section available for current flow, and hence the AC resistance increases and becomes frequency dependent until the point where almost all the current is flowing on the surface of the trace. Figure 5 shows that even at the relatively modest frequency of 100MHz the skin depth of current flowing in the conductor is only penetrating to a depth of 6 microns – at 1GHz it is down to a single micron depth – only a tiny fraction of the available conductor:
The following chart, Figure 6, shows that at around 100MHz on this structure, the overall losses are still relatively low and are split roughly 50/50 between conductor losses and dielectric losses.
Now ask yourself – what needs to happen to the copper to promote adhesion? Typically, the surface is roughened, and given that all the current is flowing on the surface of the trace, the Cu roughness adds a second hit and robs the signal of yet more energy, Figures 7 and 8:
A 5 micron RMS roughness (Figure 8) effectively doubles the amount of conductor loss at the above frequency (Figure 7), but as we journey on up the frequency band the skin effect losses, though continuing to increase, only increase in proportion to the square root of the frequency.
Dielectric loss
Dielectric losses though – which are present but at very low levels at the above frequency increase relentlessly and in direct proportion to frequency, meaning that as you move upward, the dielectric losses ultimately become the predominant loss mechanism. Dielectric loss is the heat energy lost as the substrate charges and discharges; there is a vast range of base materials available from very lossy through to ultra low loss, but regardless of the material there will be at some point a place where dielectric losses become the main loss mechanism; obviously this shift is at much higher frequencies with low loss materials.
Figure 9 shows this in graphic detail; for clarity I have removed the surface roughness effect from the graph, but you can clearly see as the frequency heads up to 100GHz that, of the 8dB per inch of loss, only 0.4 dB of the contribution is from conductor losses.
So there you have it – from DC to 100GHz – I think that is quite far enough for the moment, but if this has whet your appetite for a deeper understanding, I would thoroughly recommend the following reading:
Dr Eric Bogatin – Signal Integrity Simplified, Prentice Hall, ISBN 0-13-066946-6
Dr Howard Johnson, Dr Martin Graham – High Speed Signal Propagation – Advanced Black Magic, Prentice Hall, ISBN -13-084408-X
Stephen Hall / Garrett Hall / James A McCall – High Speed Digital System Design, John Wiley &Sons, Inc. ISBN 0-471-36090-2
More Columns from The Pulse
The Pulse: Ultra Upgrade Unknowns—What’s Coming for UHDI?The Pulse: Commonsense Cost Cutting
The Pulse: Overconstraining: Short, Slim, and Smooth
The Pulse: Drilling Down on Documentation
The Pulse: New Designer’s (Partial) Guide to Fabrication
The Pulse: Simplest Stackups Specified
The Pulse: Rough Roughness Reasoning
The Pulse: Industry Organizations Keep Knowledge Alive